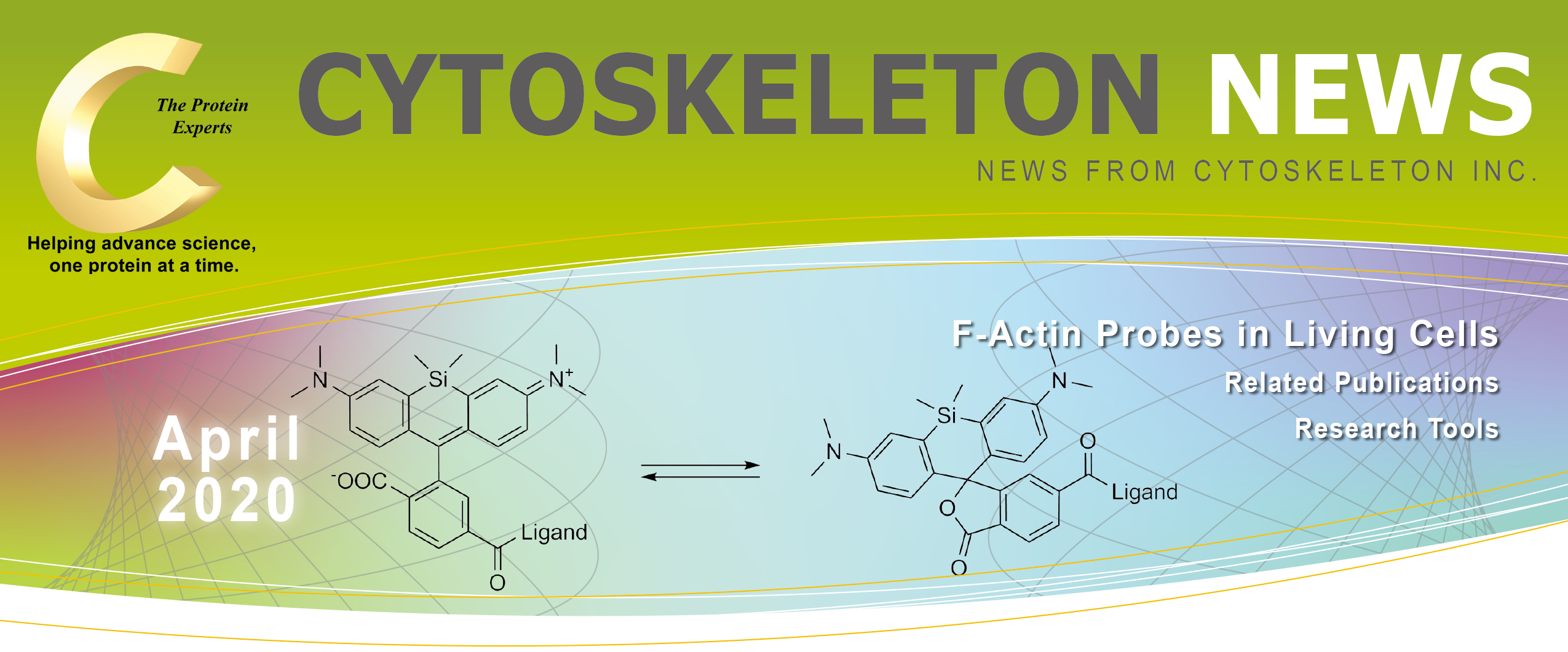
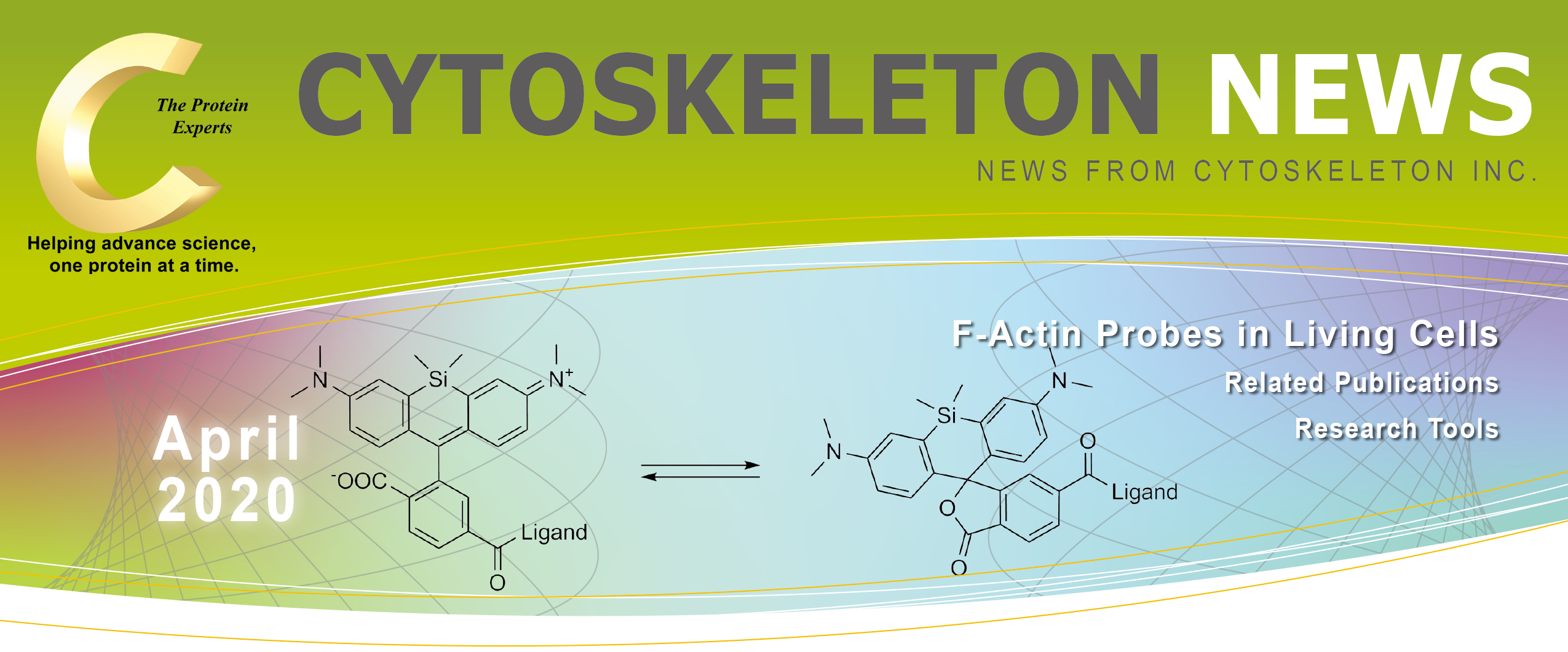
生細胞におけるF-アクチンプローブ
アクチン細胞骨格の動的なリモデリング(例:線維状アクチン(F-アクチン)と単量体アクチン(G-アクチン)間の迅速な循環)は、細胞内輸送、細胞増殖、形態、運動性、輸送、極性、細胞間接触、および細胞質分裂といった複数の生理学的機能に必須です 1, 2)。同様に、アクチン細胞骨格動態の機能不全は、発がん性、神経変性、または心血管系由来をはじめとする数々のヒト疾患の病態生理学的特徴です 3−9)。このような理由から、健常または疾患の細胞培養モデルにおけるアクチン細胞骨格動態を研究するためにF-アクチン生細胞イメージングプローブへの関心が続いています(表1)。
SiR/SPY-アクチン | GFPタグ付きLifeact | 蛍光標識アクチン | GFPタグ付きアクチン | |
---|---|---|---|---|
由来 | Jaspis johnstoni 脱臭化 脱メチル ジャスプラキノリド付加したSiRまたはSPYプローブ |
Saccharomyces cerevisiae ABP140のアミノ酸1-17 |
骨格筋(ウサギ)または非筋肉(ヒト血小板βアクチン) | βアクチンのGFPとの融合タンパク質 |
アプリケーション | 広視野共焦点などによる内在性F-アクチンの生細胞イメージング 14-18) 超解像顕微鏡(STED, SIMなど)13-18) |
生細胞イメージング 33, 34) | FRAP 50) 生細胞イメージング 51) |
FRAP 23) 外来生および内在性アクチンの生細胞イメージング 24, 25) |
利点 | 細胞や組織への直接使用可能 細胞透過性(トランスフェクションは不要) 光安定性 バックグラウンドが非常に低い 超解像に対応(STED, SIM) 細胞毒性がない 多色(近赤外、赤、燈など) F-アクチンにのみ結合 小さな有機分子(より安定) |
多色(近赤外、赤、燈、黄、緑など) | 内在性アクチンと非常に類似した立体構造 フルオフロフォアの大きさが小さい |
内在性線維に組み込まれた標識アクチン 多色(近赤外、赤、燈、黄、緑など) |
欠点 | 高濃度においてアクチン動態へ影響を及ぼす可能性 | 高分子の蛍光レポータGFP G-アクチンに結合し高いバックグラウンドシグナルを産生 アクチン動態へ影響を及ぼす可能性 トランスフェクションが必要 |
注入が必要 | 高分子の蛍光レポータGFP 外来性アクチン発現 G-アクチンに結合し高いバックグラウンドシグナルを産生 トランスフェクションが必要 |
総合評価 | +++++ | +++ | +++ | ++ |
GFPタグ付きユートロフィン | GFPタグ付きF-Tractin | GFPタグ付きナノボディ | GFPタグ付きアフィマー | |
---|---|---|---|---|
由来 | Homo sapiens ITPKAのアミノ酸10-52 |
Rattus norvegicus ITPKAのアミノ酸10-52 |
Vicugna pacos 抗-アクチン-ナノボディ |
ファージライブラリスクリーニングで単離された合成アクチン結合プローブ |
アプリケーション | 内在性F-アクチンの生細胞イメージング 41) | 内在性F-アクチンの生細胞イメージング 45, 46) | 内在性アクチンの生細胞イメージング 52) | FRAP 47-49) 内在性アクチンの生細胞イメージング 47-49) |
利点 | アクチン単量体(G-アクチン)に結合しない 多色(近赤外、赤、燈、黄、緑など) |
アクチン単量体(G-アクチン)に結合しない 多色(近赤外、赤、燈、黄、緑など) |
プローブの大きさが小さい アクチン動態への影響の可能性が低い 多色(近赤外、赤、燈、黄、緑など) |
F-アクチンに対するナノモル濃度での親和性が高い 多色(近赤外、赤、燈、黄、緑など) 正しいスクリーニングプロトコールを使えばF-アクチン特異的 |
欠点 | サイズが大きい 高分子の蛍光レポータGFP アクチン動態へ影響を及ぼす可能性 トランスフェクションが必要 |
高分子の蛍光レポータGFP アクチン動態へ影響を及ぼす可能性 トランスフェクションが必要 |
高分子の蛍光レポータGFP G-アクチンに結合し高いバックグラウンドシグナルを産生 トランスフェクションが必要 |
高分子の蛍光レポータGFP トランスフェクションが必要 |
総合評価 | +++ | +++ | +++ | +++ |
SiR と SPY アクチンプローブ
高感度かつ選択的にF-アクチンに結合できる一方で、アクチンのリモデリングを阻害しない小分子が理想的なアクチン可視化ツールとされています。さらに、トランスフェクションやエレクトロボレーションを必要とせずに培養細胞や組織に直接導入できることが、より都合がよいとされています 10-13)。新規のSiR/SPYアクチンプローブは既存のアクチンプローブにおける懸念や欠点を克服しつつ、「理想的な」アクチン結合分子のニーズを満たしています(表1、図1、2)。2014年に市販されたSiRとSiR700-アクチン生細胞イメージングプローブは、Lukinaviciusら 12, 13) によって最初に提案され、標識アクチンタンパク質やアクチン結合タンパク質のトランスフェクションや過剰発現を必要とせずに内在性のF-アクチンを標識します 12, 13) 。SiR/SiR700-アクチンプローブは、構造的に天然に存在するF-アクチン結合分子ジャスプラキノリド(jasplakinolide)に類似しています 12, 13)。これらのF-アクチンプローブは明るく光安定性に優れた近赤外色素であり、ほとんどバックグラウンドのない、独自のフルオロフォアシリコンローダミン(SiR)を利用しています(図1、2)。SiRプローブは非蛍光性、閉環のオフ状態(スピロラクトン:spirolactone)で存在するため、プローブはF-アクチンから乖離すると自己消光されます 12, 13)(図3)。SiRプローブは、広範囲で遺伝的にコードされたレポーター蛍光色素(例:GFP、mCherry)と互換性のある標準的なCy5設定(至適励起: 650nm、蛍光:670nm)で可視化することができます 12, 13)。SPY555-アクチンは、F-アクチン生細胞イメージングプローブのSpirochromeシリーズに新たに追加されました。SPY555-アクチンはSiRアクチンプローブの改良版であり、より低濃度で使用できることから頑強に標識することができ、また細胞毒性やアクチン細胞骨格ダイナミクスの摂動も低減されます。SPY555-アクチンは、SiR/SiR700-アクチンと同じ染色プロトコールを用いて標準的なTMRやCy3チャネル(最適励起: 555 nm、蛍光: 580nm)でイメージングできます。SiRとSPYアクチンプローブの主な特長は、細胞透過性、蛍光発生的特性、最小限の細胞毒性、光安定性、および標準的な蛍光顕微鏡(例えば、広視野、共焦点)と超解像顕微鏡(例えば、STED、SIM)の両方に対応することがあげられます 12-18)(図1、2)。STEDとSiR/SPY-アクチンプローブとを組み合わせて使用することで、細胞内のF-アクチン構造の比類のない蛍光可視化と生細胞に内でのそれらの物理的特性の評価を可能にします 14-18)(図1、2)。SiR-アクチンプローブは、組織内 19) やヒトiPS細胞株、心筋細胞、内皮細胞、上皮細胞、筋細胞、複数のがん細胞、および初代ニューロンといったさまざまな細胞種のF-アクチンの検討に使用されています 14, 16-18, 20-22)(図1、2)。
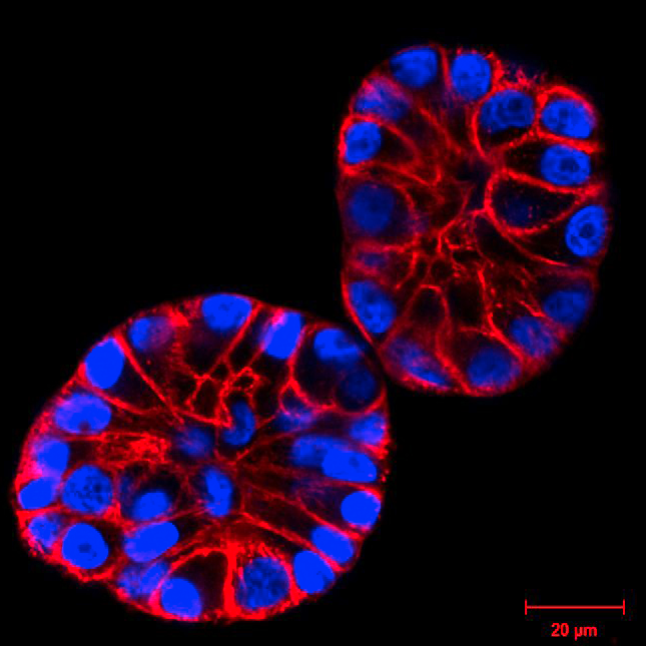
Christian Conrad と Katharina Jechow, Heidelbergよりご提供頂いた。
写真はスピノクロムウェブサイトの表紙ページのものである。
蛍光アクチンと蛍光アクチン結合ドメイン
生細胞のアクチン動態に関する最初の研究は、アクチンタンパク質の蛍光誘導体を細胞に微量注入して行われました 50, 51)。これは非常に効率的な手法ですが、装置の設定に時間を要することから、次第にGFP-アクチン抱合体をトランスフェクションする方法がより一般的になりました。蛍光標識したアクチンタンパク質やGFP/eGFP-アクチンは光褪色後蛍光回復法(FRAP)で良好に作用した 11, 23-25) ことから、アクチン細胞骨格再編成の動的性質が示されました。しかし、GFP/eGFP-アクチンにはいくつかの欠点があります 10)。第一に、GFPは分子量大きい(〜28 kDa)ため、重合化を阻害する場合があり 26)、GFP-アクチンがF-アクチン構造を特異的に標識する可能性があります 10, 24)。第二に、いくつかのアクチン結合タンパク質(例:フォルミンファミリー核形成促進因子)は、GFP-アクチンがアクチンシードや成長する重合体への組み込みを立体的に阻害する可能性があります 27, 28)。第三に、非フィラメント状蛍光アクチンから比較的高いバックグラウンドシグナルがみられることです 29)。最後に、eGFP-アクチンの発現が細胞の挙動に影響を及ぼすことがあります 30, 31)。
その他のアクチン生細胞イメージングの方法として、酵母やヒト由来アクチン結合ドメインをGFP、eGFP、またはm-Cherryフルオロフォアに結合させて使用する場合があります 10, 11, 32)。遺伝的にコードされたF-アクチンプローブで最も一般的なのは、Lifeact、ユートロフィン(UtrCH)、およびF-tractinです 10)。Lifeactは酵母Abp140由来の17アミノ酸からなるペプチド 33, 34)で、哺乳動物細胞や非哺乳動物細胞における生細胞イメージングに使用されています 24, 34-36)。Lifeactには、アクチン動態に影響を及ぼす可能性(Lifeact-induced artifactと呼ばれる)や、コフィリンなどのアクチン関連タンパク質の結合を阻害する可能性などいくつかの欠点があります 32, 37-40)。Lifeact-GFPはF-アクチンに強力に結合(Kd, 2.2 ± 0.3 μM)するものの、そのG-アクチンへの結合親和性は10倍高いため、バックグラウンドの蛍光が高くなります。Lifeactはすべてのアクチンをふくむ構造に結合するわけではありません 10, 38)。Lifeactは、SiR/SiR700/SPY プローブのように単に培地に添加するのではなく、トランスフェクションにより細胞に導入されます。UtrCHはutrophinのタンデムカルポニン相同性ドメイン(CH1とCH2)に基づいており 41)、アクチン結合タンパク質であるヒトutrophinの最初の261アミノ酸残基から構成されています 42)。CHドメインはアクチンにKd = 〜18 μMで結合します 43)。utrophinベースのプローブは広範な細胞や生物種にわたり使用されています 10, 32)。Lifeactと同様に、utrophinベースのプローブは高濃度ではアクチン細胞骨格動態に対して有害な影響を示します 32, 37, 44)。F-tractinは、ラットのアクチン結合イノシトール1,4,5-三リン酸3-キナーゼAに由来する43アミノ酸からなるペプチドであり、F-アクチンにKd = 〜10 μMで結合します 45, 46)。F-tractinは(他のプローブと比較して)よりサイズが大きいことから、重合化の調節や促進するアクチン結合タンパク質の結合を立体的に妨げる可能性 10) や、アクチンベースの細胞構造を修飾する可能性があります 32)。
アクチン配向性ナノボディとF-アクチンへのアフィマータンパク質
まとめ
参考文献
- Pollard T.D. 2016. What we know and do not know about actin. In: Jockusch B. (eds.) The Actin Cytoskeleton. Handbook of Experimental Pharmacology. vol 235. Springer, Cham.
- Dominguez R. and Holmes K.C. 2011. Actin structure and function. Annu. Rev. Biophys. 40, 169-186.
- Verstraelen P. et al. 2018. Image-based profiling of synaptic connectivity in primary neuronal cell culture. Front. Neurosci. 12, 389.
- Hoover B.R. et al. 2010. Tau mislocalization to dendritic spines mediates synaptic dysfunction independently of neurodegeneration. Neuron. 68, 1067-1081
- Spires-Jones T.L. and Hyman B.T. 2014. The intersection of amyloid beta and tau at synapses in Alzheimer’s disease. Neuron. 82, 756-771.
- Dugina V.B. et al. 2019. Biological role of actin isoforms in mammalian cells. Biochemistry (Mosc). 84, 583-592.
- Hartmann S. et al. 2015. The function of Rho-associated kinases ROCK1 and ROCK2 in the pathogenesis of cardiovascular disease. Front. Pharmacol. 6, 276.
- Bonello T.T. et al. 2009. New approaches to targeting the actin cytoskeleton for chemotherapy. Future Med. Chem. 1, 1311-1331.
- Bryce N.S. et al. 2019. Chemical biology approaches targeting the actin cytoskeleton through phenotypic screening. Curr. Opin. Chem. Biol. 51, 40-47.
- Melak M. et al. 2017. Actin visualization at a glance. J. Cell Sci. 130, 525-530.
- Belin B.J. et al. 2014. Comparative analysis of tools for live cell imaging of actin network architecture. Bioarchitecture. 4, 189-202.
- Lukinavicius G. et al. 2013. A near-infrared fluorophore for live-cell super-resolution microscopy of cellular proteins. Nat. Chem. 132-139.
- Lukinavicius G. et al. 2014. Fluorogenic probes for live-cell imaging of the cytoskeleton. Nat. Methods. 11, 731-733.
- D’Este E. et al. 2015. STED nanoscopy reveals the ubiquity of subcortical cytoskeleton periodicity in living neurons. Cell Rep. 10, 1246-1251.
- Hell S.W. and Wichmann J. 1994. Breaking the diffraction resolution limit by stimulated emission: stimulated-emission-depletion fluorescence microscopy. Opt. Lett. 19, 780-782.
- Bar J. et al. 2016. Periodic F-actin structures shape the neck of dendritic spines. Sci. Rep. 6, 37136.
- Liete S.C. et al. 2016. The actin-binding protein α-adducin is required for maintaining axon diameter. Cell Rep. 15, 490-498.
- Wang L. et al. 2019. Small-molecule fluorescent probes for live-cell super-resolution microscopy. J. Am. Chem. Soc. 141, 2770-2781.
- Klementieva N.V. et al. 2016. Fluorescence imaging of actin fine structure in tumor tissues using SiR-actin staining. Anticancer Res. 36, 5287-5294.
- Kim T.H. et al. 2016. Cancer cells become less deformable and more invasive with activation of β-adrenergic signaling. J. Cell Sci. 129, 4563-4575.
- Hashimoto N. et al. 2017. Reversible differentiation of immortalized human bladder smooth muscle cells accompanied by actin bundle reorganization. PLoS ONE. 12, e0186584.
- Narva E. et al. 2017. A strong contractile actin fence and large adhesions direct human pluripotent colony morphology and adhesion. Stem Cell Reports. 9, 67-76.
- Choidas A. et al. 1998. The suitability and application of a GFP-actin fusion protein for long-term imaging of the organization and dynamics of the cytoskeleton in mammalian cells. Eur. J. Cell Biol. 77, 81-90.
- Lemieux M.G. et al. 2014. Visualization of the actin cytoskeleton: different F-actin-binding probes tell different stories. Cytoskeleton. 71, 157-169.
- Small J. et al. 1999. Visualising the actin cytoskeleton. Microsc. Res. Tech. 47, 3-17.
- Sliogeryte K. et al. 2016. Differential effects of LifeAct-GFP and actin-GFP on cell mechanics assessed using micropipette aspiration. J. Biomech. 49, 310-317.
- Wu J.-Q. and Pollard T.D. 2005. Counting cytokinesis proteins globally and locally in fission yeast. Science. 310, 310-314.
- Chen Q. et al. 2012. Formins filter modified actin subunits during processive elongation. J. Struct. Biol. 177, 32-39.
- Lee C.W. et al. 2013. Dynamic localization of G-actin during membrane protrusion in neuronal motility. Curr. Biol. 23, 1046-1056.
- Aizawa H. et al. 1997. A green fluorescent protein-actin fusion protein dominantly inhibits cytokinesis, cell spreading, and locomotion in Dictyostelium. Cell Struct. Funct. 22, 335-345.
- Nagasaki A. et al. 2017. The position of the GFP tag on actin affects the filament formation in mammalian cells. Cell Struct. Funct. 42, 131-140.
- Patel S. et al. 2017. Functional characterisation of filamentous actin probe expression in neuronal cells. PLoS ONE. 12, e0187979.
- Riedl J. et al. 2008. Lifeact: a versatile marker to visualize F-actin. Nat. Methods. 5, 605-607.
- Riedl J. et al. 2010. Lifeact mice for studying F-actin dynamics. Nat. Methods. 7, 168-169.
- Schachtner H. et al. 2012. Tissue inducible Lifeact expression allows visualization of actin dynamics in vivo and ex vivo. Eur. J. Cell Biol. 91, 923-929.
- Mizoguchi T. et al. 2016. Zebrafish lines expressing UAS-driven red probes for monitoring cytoskeletal dynamics. Genesis. 54, 483-489.
- Du J. et al. 2015. Lifeact and Utr230 induce distinct actin assemblies in cell nuclei. Cytoskeleton. 72, 570-575.
- Munsie L.N. et al. 2009. Lifeact cannot visualize some forms of stress-induced twisted F-actin. Nat. Methods. 6, 317.
- Courtemanche N. et al. 2016. Avoiding artefacts when counting polymerized actin in live cells with LifeAct fused to fluorescent proteins. Nat. Cell Biol. 18, 676-683.
- Flores L.R. et al. 2019. Lifeact-TagGFP2 alters F-actin organization, cellular morphology and biophysical behaviour. Sci. Rep. 9, 3241.
- Burkel B.M. et al. 2007. Versatile fluorescent probes for actin filaments based on the actin-binding domain of utrophin. Cell Motil. Cytoskelet. 64, 822-832.
- Winder S.J. et al. 1995. Calmodulin regulation of utrophin actin binding. Biochem. Soc. Trans. 23, 397S.
- Rybakova I.N. and Ervasti J.M. 2005. Identification of spectrin-like repeats required for high affinity utrophin-actin interaction. J. Biol. Chem. 280, 23018-23023.
- Spracklen A.J. et al. 2014. The pros and cons of common actin labeling tools for visualizing actin dynamics during Drosophila oogenesis. Dev. Biol. 393, 209-226.
- Schell M.J. et al. 2001. Inositol 1,4,5-triphosphate 3-kinase A associates with F-actin and dendritic spines via its N-terminus. J. Biol. Chem. 276, 37537-37546.
- Brehm M.A. et al. 2004. Identification of the actin-binding domain of Ins(1, 4, 5)P3 3-kinase isoform B (IP3K-B). Biochem. J. 382, 353-362.
- Lopata A. et al. 2018. Affimer proteins for F-actin: novel affinity reagents that label F-actin in live and fixed cells. Sci. Rep. 8, 6572.
- Tiede C. et al. 2014. Adhiron: a stable and versatile peptide display scaffold for molecular recognition applications. Protein. Eng. Des. Sel. 27,145-155.
- Tiede C. et al. 2017. Affimer proteins are versatile and renewable affinity reagents. Elife 6, 24903.
- Shimada Y. et al. 1997. Dynamics of actin in cardiac myofibrils and fibroblast strees fibers. Cell Structure and function, 22, 59-64.
- Machesky L.M. and Hall A. 1997. Role of actin polymerization and adhesion to extracellular matrix in Rac- and Rho-induced cytoskeletal reorganization. J. Cell Biol. 138, 913-926.
- Rocchetti A. et al. 2014. Fluorescent labelling of the actin cytoskeleton in plants using a cameloid antibody. Plant Methods 10, 12 10.1186/1746-4811-10-12.
Live Cell Imaging Products
品名 | メーカー | 品番 | 包装 | 希望販売価格 |
---|---|---|---|---|
SPY555-Actin Probe![]() |
CYT | CY-SC202 | 1 VIAL [100 slides] |
¥158,000 |
SPY505-DNA Probe![]() |
CYT | CY-SC101 | 1 VIAL [100 slides] |
¥119,000 |
SPY555-DNA Probe![]() |
CYT | CY-SC201 | 1 VIAL [100 slides] |
¥119,000 |
SPY555-Tubulin Probe![]() |
CYT | CY-SC203 | 1 VIAL [100 slides] |
¥158,000 |
SPY595-DNA Probe![]() |
CYT | CY-SC301 | 1 VIAL [100 slides] |
¥119,000 |
SPY650-DNA Probe![]() |
CYT | CY-SC501 | 1 VIAL [100 slides] |
¥119,000 |
SPY650-Tubulin Probe![]() |
CYT | CY-SC503 | 1 VIAL [100 slides] |
¥158,000 |
SPY700-DNA Probe![]() |
CYT | CY-SC601 | 1 VIAL [100 slides] |
¥119,000 |
SiR-Actin Kit![]() |
CYT | CY-SC001 | 1 KIT [50-300 slides] |
¥180,000 |
SiR-Tubulin Kit![]() |
CYT | CY-SC002 | 1 KIT [50-300 slides] |
¥180,000 |
Cytoskeleton Kit (SiR-Actin + SiR-Tubulin)![]() |
CYT | CY-SC006 | 1 KIT [50-300 slides] |
販売終了 |
SiR-DNA Kit![]() |
CYT | CY-SC007 | 1 KIT [50-300 slides] |
¥119,000 |
SiR700-Actin Kit![]() |
CYT | CY-SC013 | 1 KIT [35-200 slides] |
¥180,000 |
SiR700-Tubulin Kit![]() |
CYT | CY-SC014 | 1 KIT [35-200 slides] |
¥180,000 |
SiR700-DNA Kit![]() |
CYT | CY-SC015 | 1 KIT [35-200 slides] |
¥119,000 |
ActiStain™ Phalloidins
品名 | メーカー | 品番 | 包装 | 希望販売価格 |
---|---|---|---|---|
Acti-stainTM 488 phalloidin, Plant![]() |
CYT | PHDG1-A | 1*500 UL [300 slides] |
¥74,000 |
Acti-stainTM 555 phalloidin, Mushroom![]() |
CYT | PHDH1-A | 1*500 UL [300 slides] |
¥74,000 |
Acti-stainTM 670 phalloidin, Plant![]() |
CYT | PHDN1-A | 1*500 UL [300 slides] |
¥74,000 |
Phalloidin; Fluorescent Derivatives (Acti-StainTM 535), Rhodamine Isothiocyanate![]() |
CYT | PHDR1 | 1*500 UL [300 slides] |
¥74,000 |
Labeled Actin Proteins
品名 | メーカー | 品番 | 包装 | 希望販売価格 |
---|---|---|---|---|
Actin Protein (rhodamine, platelet non-muscle), Human, Rhodamine Isothiocyanate![]() |
CYT | APHR-A | 4*10 UG |
¥101,000 |
Actin Protein (rhodamine, platelet non-muscle), Human, Rhodamine Isothiocyanate![]() |
CYT | APHR-C | 20*10 UG |
¥321,000 |
Actin Protein (rhodamine, skeletal muscle), Rabbit, Rhodamine Isothiocyanate![]() |
CYT | AR05-B | 10*20 UG |
¥98,000 |
Actin Protein (rhodamine, skeletal muscle), Rabbit, Rhodamine Isothiocyanate![]() |
CYT | AR05-C | 20*20 UG |
¥189,000 |
Actin Biochem Kits
品名 | メーカー | 品番 | 包装 | 希望販売価格 |
---|---|---|---|---|
Actin Binding Protein Spin-down Biochem Kit (for muscle)![]() |
CYT | BK001 | 1 KIT [30-100 assays] |
¥254,000 |
Actin Polymerization Biochem Kit, Rabbit![]() |
CYT | BK003 | 1 KIT [30-100 assays] |
¥288,000 |
Actin Binding Protein Spin-Down Biochem Kit (Non-muscle)![]() |
CYT | BK013 | 1 KIT [30-100 assays] |
¥257,000 |
G-Actin : F-Actin In Vivo Assay Kit, Mouse![]() |
CYT | BK037 | 1 KIT [30-100 assays] |
¥250,000 |
G-LISA Activation Assay Kits
品名 | メーカー | 品番 | 包装 | 希望販売価格 |
---|---|---|---|---|
RhoA G-LISA(R) Activation Assay (luminescence)![]() |
CYT | BK121 | 96 ASSAY |
¥302,000 |
RhoA G-LISA(R) Activation Assay (absorbance)![]() |
CYT | BK124 | 96 ASSAY |
¥302,000 |
Rac1,2,3 G-LISA(R) Activation Assay Kit![]() |
CYT | BK125 | 96 ASSAY |
¥302,000 |
Rac1 G-LISA(R) Activation Assay Kit![]() |
CYT | BK126 | 1 KIT [96 assays] |
¥302,000 |
Rac1 G-LISA(R) Activation Assay Kit![]() |
CYT | BK128 | 1 KIT [96 assays] |
¥302,000 |
Ras G-LISA(R) Activation Assay Kit![]() |
CYT | BK131 | 1 KIT [96 assays] |
¥302,000 |
RhoA ELISA Kit![]() |
CYT | BK150 | 1 KIT [96 assays] |
¥169,000 |
Pull Down Activation Assay Kits
品名 | メーカー | 品番 | 包装 | 希望販売価格 |
---|---|---|---|---|
Ras Activation Assay Biochem Kit![]() |
CYT | BK008 | 1 KIT [50 assays] |
¥269,000 |
Combo RhoA/Rac1/Cdc42 Activation Assay Kit![]() |
CYT | BK030 | 1 KIT [3 x 10 assays] |
¥327,000 |
Cdc42 Pulldown Activation Assay Kit![]() |
CYT | BK034 | 1 KIT [50 assays] |
¥269,000 |
RhoA Pulldown Activation Assay Kit![]() |
CYT | BK036 | 1 KIT [80 assays] |
¥269,000 |
■ CYTOSKELETON NEWS バックナンバー
- 2020年10月号 紡錘体 - 可視化に向けた新規ツール
- 2020年8月号 細胞膜染色用蛍光プローブ
- 2020年4月号 生細胞におけるF-アクチンプローブ
- 2020年3月号 コロナウイルスと細胞骨格
- 2020年2月号 タウ(Tau)の将来性をMapping
- 2020年1月号 Rho GTPaseによる細胞遊走制御
- 2019年12月号 表現型プロファイリング:アクチンに焦点を当てたがん治療
- 2019年11月号 チューブリンの過剰グルタミル化、ミトコンドリア、神経変性
- 2019年9月号 細胞運動性を制御するために相互作用する膜張力とアクチン細胞骨格
- 2019年8月号 Rac1B、がん、およびRac1
- 2019年7月号 Rhoファミリー GTPases、神経可塑性、およびうつ状態
- 2019年6月号 アクチンメチオニン酸化: 動的制御の次の段階
- 2019年5月号 ミクログリアと神経変性疾患
- 2019年2月号 生細胞画像化に対するCNS疾患や障害
2018年
- 2018年12月号 アクチン細胞骨格とメカノトランスダクション(機械的シグナル伝達)
- 2018年11月号 軸索再生と細胞骨格
- 2018年10月号 ニューロンにおける微小管と極性
- 2018年8月号 Rab GTPase と 神経変性
- 2018年7月号 SUMO レスリング: バランスが全て
- 2018年6月号 なぜ K-Ras は発がん特異性を示すのか?
- 2018年5月号 治療標的としてのユビキチンプロテアソームシステム:チューブリンは関与するか?
- 2018年4月号 RhoファミリーGEFと樹状突起スパインの構造的可塑性
- 2018年3月号 βカテニンとTFC/LEF-1の翻訳後修飾による標準的なWntシグナル制御
- 2018年2月号 がん抑制遺伝子p53の翻訳後修飾による機能の調整
- 2018年1月号 自閉スペクトラム症におけるGEF Trioの役割
2017年
- 2017年12月号 プロフィリン: アクチン結合タンパク質の多機能な役割
- 2017年11月号 ミトコンドリアにおけるアセチル化:新たな考え方と治療への応用の可能性
- 2017年9月号 翻訳後修飾のアセチル化による微小管の安定化
- 2017年8月号 神経軸索におけるアクチンリングを基盤とした周期的膜骨格(PMS)
- 2017年7月号 E3ユビキチンリガーゼMdm2によるがん抑制遺伝子p53の翻訳後制御
- 2017年6月号 多能性幹細胞(PSC)での転写因子による翻訳後制御
- 2017年5月号 Arf6 GEF と癌細胞の浸潤・転移
- 2017年4月号 PTEN(Phosphatase and Tensin Homolog)による翻訳後制御
- 2017年3月号 Tau の翻訳後修飾: アルツハイマー病の治療標的
- 2017年2月号 樹状細胞の移動におけるアクチン結合タンパク質とF-アクチン
2016年
- 2016年11月/12月号 GEF を介した GTPase シグナル伝達の低分子阻害剤
- 2016年9月号 FtsZ タンパク質: 抗菌薬の新規ターゲット
- 2016年7月号 翻訳後修飾(PTM)は心臓病において細胞骨格タンパク質を調節する
- 2016年6月号 モータータンパク質キネシンと神経変性
- 2016年5月号 チロシンリン酸化は Rhoファミリー GTPase 活性を調節する
- 2016年4月号 Rac1と糖尿病: ポジティブな役割とネガティブな役割
- 2016年3月号 SUMO化: 細胞骨格タンパク質の機能を調節するレギュレーター
- 2016年1月/2月号 ビメンチン中間径フィラメント: リン酸化による調節
2015年
- 2015年8月号 タンパク質調節に不可欠な翻訳後修飾
- 2015年7月号 アクチン細胞骨格のライブセルイメージング
- 2015年6月号 有糸分裂に関わるタンパク質のSUMO化: 局在と機能
- 2015年5月号 Ras 癌の治療: 5つの有望なターゲット
- 2015年4月号 Ras 依存性の癌で注目される YAP1
- 2015年3月号 増刊号 統合失調症において遺伝子変異により誘導されるアクチン依存のシナプスの変化
- 2015年3月号 Ral GTPase を調節する翻訳後修飾
- 2015年1月/2月号 RhoA のリン酸化はシグナル伝達を調節する
- 2015年1月号 増刊号 微小管を不安定化する suprafenacine: 新規抗癌剤のリード化合物としての可能性
2014年
- 2014年12月号 増刊号 RhoA は心筋細胞におけるアクチン細胞骨格の再構成とグルコース取り込みを仲介する
- 2014年11月号 増刊号 樹状突起の形態形成: ドーパミンD1受容体 および Rho ファミリー GTPase による制御
- 2014年11月/12月号 GTPase 活性化アッセイ: アイソフォームの検出
- 2014年10月号 アルギニンの正電荷を消失させるシトルリン化
- 2014年9月号 キネシンサブドメインの探索
- 2014年9月号 増刊号 アクチン結合タンパク質コフィリンの S-ニトロシル化: 細胞移動に対する影響
- 2014年8月号 増刊号 原発性硬化性胆管炎における N-Ras 発現および活性
- 2014年8月号 SUMO化: 細胞骨格タンパク質を標的とした翻訳後修飾
- 2014年7月号 Sos/K-Ras 結合を介して Ras シグナル伝達を制御する新しい低分子阻害剤
- 2014年6月号 増刊号 頭頸部扁平上皮癌における microRNA-138 による RhoC のダウンレギュレーション
- 2014年6月号 Rho GTPase と活性酸素種: クロストークとフィードバック
- 2014年5月号 ミオシンのアセチル化はサルコメアの構造と機能を調節する
- 2014年4月号 リジンのアセチル化 - 多様な細胞プロセスの制御因子
- 2014年3月号 インテグリンを介したβ-アクチンの酸化還元制御: PDIの出現
- 2014年1/2月号 ダイニン: 一つのモーターが関わる複数の神経変性疾患
2013年
- 2013年11/12月号 ダイニン:チームとして強力に作用するモータータンパク質
- 2013年10月号 神経変性:Rhes、SUMO化、ハンチントン病
- 2013年9月号 モノユビキチン化:タンパク質調節のダイナミックなタグ
- 2013年8月号 Ras及びRhoのプレニル化による翻訳後修飾:癌創薬における役割
- 2013年7月号 アクチンが引き起こす膜突起による浸潤:コルタクチン
- 2013年6月号 アクチン修飾と細胞骨格
- 2013年5月号 微小管内部の実体
- 2013年4月号 神経変性におけるTauの多面性
- 2013年3月号 蛍光フィブロネクチンタンパク質を用いた特発性肺線維症の創薬
- 2013年1/2月号 樹状突起棘:発生におけるArf6の役割
2012年
- 2012年11/12月号 ミオシンの小分子モジュレーター
- 2012年10月号 Rhoファミリーパスウェイのユビキチン化と制御
- 2012年9月号 神経変性におけるRac1 GTPaseの機能
- 2012年8月号 上皮間葉転換(EMT)とRhoファミリー低分子量G-タンパク質の関与
- 2012年7月号 チューブリンの多重修飾:グルタミル化とグリシル化
- 2012年6月号 細胞接着のフィブロネクチン制御と原線維形成
- 2012年5月号 アクチン酸化サイクルの機能
- 2012年4月号 トラフィッキング:ArfとCdc42/Racの結合
- 2012年3月号 G-LISAを用いた心臓研究: 糖尿病性心筋症におけるRho経路に関する研究
- 2012年1月/2月号 FtsZ: 新たな抗生剤の標的となるチュ−ブリンホモログ
商品は「研究用試薬」です。人や動物の医療用・臨床診断用・食品用としては使用しないように、十分ご注意ください。
※ 表示価格について